Draft Development Strategy, National Water Management Plan
Arsenic in Annex C-8
C8.1.1 History, Discovery and Causes
In the last few years it has been recognized that
Bangladesh is affected by one of the worst cases groundwater contamination in
the world. With the exception of diarrheal diseases and coastal salinity in
rivers, until recently water quality was not perceived as a problem in
Bangladesh, as may be recognized by reference to the earlier National Water
Plan projects (MPO, 1986 and 1991). Medical evidence of arsenic poisoning was
first discovered in West Bengal in 1978, although
the connection with groundwater contamination was not recognized until some
years later. Further surveys were carried out during the 1980s (eg Chakraborty
et al, 1987; Mazumder et al, 1988), culminating a national
report by PHED, India, in 1991. In 1993, DPHE, Bangladesh, tested for and
identified arsenic at Chapai Nawabganj, near the border with West Bengal, but
it was not until 1995 that the existence of arsenic in Bangladesh became
widely known. It was not appreciated until early 1997 that arsenic
contamination extended over large parts of the country. By the end of 1998, a
national assessment of the extent of contamination across the country had been
made (DPHE 1999). At around the same time, projects of both the Ministry of
Heath and the DPHE as well as various NGO's began work on extensive testing,
awareness raising and providing small-scale arsenic removal systems.
C8.1.2 Studies on the Distribution and Origin of Arsenic in
Groundwater
The studies of arsenic occurrence in Bangladesh and West
Bengal may be divided into two groups, those with a medical bias aimed at
confirming diagnoses of arsenicosis and those with a hydrogeological bias
aimed at estimating exposure and the causes of contamination. The following
discussion concerns the theories about the origin of arsenic, which have
important implications for water management policy. The principal competing
explanations all point to a geological source. These ideas have been
presented both in reports and scientific literature, and are given a
detailed discussion below. Other, anthropogenic, sources have been proposed,
but have generally been made in the popular press rather than in reports or
in scientific journals.
C8.1.2.1Geological Source Hypotheses
The first major investigation of arsenic contamination in
West Bengal by PHED (1991) contained much analytical and descriptive detail
but did not suggest an origin for the contamination other than ruling out
anthropogenic sources. In the absence of any formal explanation, a number of
(non-hydogeological) studies (eg Das et al, 1994 and 1996; Chaterjee et
al, 1995) went onto suggest that extensive seasonal pumping of groundwater
for irrigation is responsible, although they did not put forward any direct
evidence to support the idea. This idea came to be known as the ‘pyrite
oxidation’ hypothesis and was formally described by Mallick and Rajagopal
(1996), although again unsupported by field evidence. The idea is based on the
assumption that arsenic is present in the sulphide minerals
pyrite and arsenopyride. According to the theory, lowering of the
water table due to pumping introduces oxygen, which causes the
breakdown of pyrite and releases arsenic, iron and sulphate into the water.
Recharge during the subsequent monsoon then flushes the arsenic into the
underlying aquifers. This explanation, whereby the arsenic pollution is caused
by man’s over-exploitation of groundwater, became well known and led to
calls for the banning of tulle well irrigation.
In 1997, an alternative explanation was independently put
forward by an Indo - Swedish group (Bhattacharya et al, 1997) and an
Anglo - Bangladeshi group (Nickson 1997; Nickson et al, 1998 and 2000)
and has become known as the 'oxyhydroxide reduction' hypothesis. Both these
groups presented their ideas in international scientific journals where the
origin of arsenic was the main subject of the papers. According to the theory,
arsenic weathered from the hard rock areas of India is carried in the suspended
load of the rivers adsorbed onto iron oxides or hydroxides. Following deposition
of this fine sediment, decomposition of organic matter leads to strongly
reducing groundwater conditions which causes dissolution of the iron
oxyhydroxides and consequent release of arsenic into solution. This hypothesis
also accounts for the pervasive occurrence of iron in wells beneath the
floodplains.
The debate between these views hinges on a number of issues.
First, the chemistry of the affected water points towards strongly reducing
conditions and hence that release by oxidation is very unlikely. Evidence for
reducing conditions includes direct measurement of redox potential,
low-to-negligible concentrations 0£ dissolved oxygen nitrate and sulphate
combined with high concentrations of iron, manganese and bicarbonate plus the
occurrence of dissolved gases such as carbon dioxide and methane (Ahmed et al,
l998). Only limited evidence has been presented for the occurrence of
arsenopyrite or arsenin-rich pyrite, while Nickson et
al (1998) showed that pryrite is present in a stable diagenetic (framboidal)
form. This indicates that it has grown after deposition and is a sink rather
than a source for arsenic. Imam et al (1998) identified the ubiquitous
presence of iron-rich coatings on sands from the affected aquifers. Further,
PHED (1991) showed very high arsenic concentrations (2000mg/kg) in the
ferruginous coatings of aquifer sands. Chemical analyses of sediments from the
arsenic affected aquifers show a good correlation between iron and arsenic but
no correlation between sulphur and arsenic. In addition, arsenic concentrations
are low at or immediately below the zone of water table fluctuation. The
combination of reducing conditions, the problem of accounting for the absence of
sulphur, and the near-neutral pH all point strongly towards the oxyhydroxide
reduction hypothesis as being the predominant mechanism of
arsenic release in the Bengal Basin.
Subsequent field and laboratory investigations have all
confirmed the ‘oxyhydroxide reduction’ hypothesis. These studies include
investigations in Meherpur by Burren (1998) and Perrin (1998), in Jessore by
AAN (1999) and in Noakhali by Mather (1999), as well as the major national
investigation by DPHE (1999). In addition, there have been two published
discussion on the 'oxyhydroxyde reduction' explanation in although again unsupported by field evidence. The idea is
based on the assumption that arsenic is present in the sulphide minerals pyrite
and arsenopyride. According to the theory, lowering of the
water table due to pumping introduces oxygen, which
causes the breakdown of pyrite and releases arsenic, iron and sulphate into the
water. Recharge during the subsequent monsoon then flushes the arsenic into the
underlying aquifers. This explanation, whereby the arsenic pollution is caused
by man’s over-exploitation of groundwater, became well known and led to calls
for the banning of tulle well irrigation. In 1997, an alternative explanation was independently put
forward by an Indo - Swedish group (Bhattacharya et al, 1997) and an
Anglo - Bangladeshi group (Nickson 1997; Nickson et al, 1998 and 2000)
and has become known as the 'oxyhydroxide reduction' hypothesis. Both these
groups presented their ideas in international scientific journals where the
origin of arsenic was the main subject of the papers. According to the theory,
arsenic weathered from the hard rock areas of India is carried in the suspended
load of the rivers adsorbed onto iron oxides or hydroxides. Following deposition
of this fine sediment, decomposition of organic matter leads to strongly
reducing groundwater conditions which causes dissolution of the iron
oxyhydroxides and consequent release of arsenic into solution. This hypothesis
also accounts for the pervasive occurrence of iron in wells beneath the
floodplains.
Other studies that contain relevant data but do not take a
strong position regarding the origin of arsenic in water include Ghosh and De
(1995), Nag et at (1996), NRECA (1997), Safiullah (1998), Talukder (1998)
and Johnston (1998). Other hydrogeological and/or geochemical studies are
underway by the US Geological Survey, the Lamont-Doherty Earth Observatory and
the Massachusetts Institute of Technology (MIT). From correspondence with these
groups, no major revision of the presently accepted (oxyhydroxide reduction)
explanation is expected, although clarification of the mechanisms and rates of
the processes can be expected.
Public interest in the origin of arsenic contamination has
important practical implications if there is a relationship between tube well
irrigation and arsenic. From the process perspective, the oxyhydroxide reduction
hypothesis is totally inconsistent with the idea that arsenic pollution is
caused by water table lowering due to irrigation pumping. In fact, that idea
suggests that lowering the water table will have the effect of removing arsenic
from shallow groundwater, an idea that is supported by evidence from dug wells
(see below). DPHE (1999) carried out statistical tests for an abstraction
between the intensity of arsenic contamination and both groundwater abstraction
for irrigation and the seasonal depth to the water table. They found significant
negative correlations with both parameters. This finding is consistent with the
simple observation that arsenic is absent in the intensively - irrigated Bogra
region and the pervasive contamination in the modestly - irrigated (by
groundwater) Chandpur - Noakhali region. While it would be unjustified to
suggest that pumping has no effect on arsenic, it can be stated confidently that
there is no evidence pointing to a causal relationship between tube well
irrigation and the occurrence of arsenic in groundwater.
As a minor modification to the above explanation, Acharyya
et al (1999), while accepting oxyhydroxide reduction as the dominant
mechanism, have suggested that phosphatic fertilizers might enhance mobilization
of arsenic in the soil zone by displacing arsenic from adsorption sites.
However, they have presented no evidence to support this, and it is clear that
even if correct, the effect would be minute compared to the quantity of arsenic
released by natural processes.
C8.1.2.2 Anthropogenic Hypotheses
Newspaper and magazine articles have suggested various
manmade sources of arsenic such as fertilizers, pesticides, wood
preservatives and even acid-mine drainage or interventions such as the
construction of barrages on regional rivers. None of these ideas have been
presented in reports or journals where a process of peer-review is possible,
and none has gained support amongst the scientific community. Virtually all
have been presented without verifiable supporting evidence and hence should
be classified as unsubstantiated speculations. Nevertheless some have gained
attention in the popular media and therefore cannot be ignored. The
wood-preservative suggestion was formally investigated by NRECA (1997) who
concluded that there was no connection.
As noted above, the chemistry of arsenic and phosphate
are related. The geographical distribution of phosphate and arsenic are
similar. However, it is more likely that phosphate and arsenic share a
common or closely related origin than that one causes the other. The
hypothesis of a causal relationship with fertilizer use is strongly opposed
by fire the virtual absence of phosphate in the intensively irrigated
Bogra-Dinajpur and Gazipur-Mymensingh regions. The similar rare occurrence
of nitrate in groundwater, especially in arsenic-affected areas, also argues
against widespread fertilizer pollution.
Claims for connection between barrage building and
arsenic contamination are highly tenuous, requiring pyrite oxidation as a
mechanism, which as discussed above is rejected by most workers. It is
claimed that arsenic poisoning began after the opening of the Farraka
barrage. The absence of diagnosed patients is almost certainly due to the
absence of testing. Further, with the first diagnosis in 1978 and a lag time
of five to 15 years, this points to contamination being present at least in
the 1960s. In addition, DPHE (1999) argue geologically that contamination is
present mainly in aquifer sediments deposited in the last 18,000 years.
Confirming this, the International Atomic Energy Authority have carried out
radio-carbon dating on arsenic-contaminated groundwater which points to
residence-times of a few hundred to a few thousands years. Moreover, the
regional pattern of contamination is inconsistent with such a cause. For
instance, whatever impacts Farraka may have had on the South - West region,
it is inconceivable that it could have had a major impact on groundwater
levels in the north of the Sylhet Basin.
All of these anthropogenic hypotheses face profound
difficulties in explaining the observed geographical occurrence (and
absence) of arsenic in groundwater, and all lack supporting evidence. These
hypotheses are rejected as a basis for strategy development.
C.8.2 Safe Levels of Arsenic in Drinking Water
Arsenic in the environment may exist in a number of
organic and inorganic forms. The most toxic form of arsenic is arsine gas,
followed by arsenite and arsenate
which are the forms of arsenic normally found in groundwater.
Organic forms of arsenic such as found in many foodstuffs are much less toxic
(Morton and Dunnette, 1994; FDA, 1993) than the inorganic forms in drinking
water.
Arsenic is both toxic and carcinogenic (Morton and Dunnette,
1994). The clinical effects of chronic arsenic
poisoning range from skin ailments (keratosis and melanosis), through damage
to internal organs to gangrene and various forms of cancer. The clinical
effects of arsenic in drinking water in Bangladesh have been extensively
demonstrated by Dhaka Community Hospital and NIPSOM amongst others.
The present drinking water standard adopted in Bangladesh and
India is 0.05mg/l (50ppb) of (total) arsenic, which followed WHO advice at the
time the standards were set. In 1993, the WHO proposed a new provisional
guideline value for arsenic of 0.01mg/l (10ppb). This limit is based on
practical limits of detection of arsenic and not on the normal health
risk criteria. Conventionally, limits for carcinogens are set at
the level of one excess lifetime cancer per 10,000 of population exposed. The
provisional value of 0.01mg/l corresponds to an estimated risk of six additional
skin cancers per 10,000 exposed persons.
The European Union has adopted the guideline value of
0.01mg/l, while Heath Canada has adopted an interim maximum acceptable
concentration of 0.025mg/l (25ppb). The USA still works to a standard 0.05mg/l,
but this year (2000) the US EPA has proposed that the standard should be reduced
to 0.005mg/l (5ppb). This is expected to become law within a year. The US EPA
also proposes a Health Goal (the level of no known or anticipated health
effects) of zero.
It is anticipated that there will be demands to lower the
standard for acceptable concentrations of arsenic
in drinking water m Bangladesh. However, in considering the implications of tbe
arsenic drinking water standard, it is important that the intervention
strategies consider the dose-response relationships. As
indicated above, the number of cases of arsenicosis resulting
from drinking water at the current standard is only a small proportion of the
exposed population. However, published dose-response curves from China (Lianfang
and Jianzhong, 1994) suggests that the prevalence rate of arsenicosis rises to
10% at about 0.4mg/l, and to the order of 40% of the exposed population at
concentrations of around 0.6mg/1. As discussed below, such concentrations are
not rare in Bangladesh.
In summary, epidemiological evidence from around the world
indicates that the present Bangladesh Drinking Water Standard does not
correspond to a safe level according to the standard criteria of WHO and others.
On the other hand, the dose - response function makes it imperative that
interventions are prioritized according to the concentrations to which people
are exposed.
C8.3 Occurrence of Arsenic
C8.3.l Testing for Arsenic
The most reliable determination of arsenic in groundwater is carried out by
analyzing filtered and acidified samples in a laboratory using an atomic
adsorption spectrometer. However, the scale of the problem in Bengal calls for
a field test kit. Field kits, however, have often been criticized for being
unreliable. In addition, DPHE (1999) have demonstrated the unreliability and
lack of quality control in many laboratories in Bangladesh. However, DPHE
(1999) also demonstrated that any error consistently underestimates the true
level of arsenic, and that field - kits can provide valid regional
descriptions of arsenic occurrence.
The practical detection limits of field
- kits are close to the present drinking water standard of 0.05mg/l, and
become unreliable for waters close to this value. Importantly, field kits do
reliably identify highly contaminated wells. However, if a lower drinking
water standard is adopted, the present field kits will not be able to
differentiate between safe and unsafe water. The only field method applicable
at these concentrations is a prototype field
- kits known as the “Arsenator”.
The most comprehensive, systematic and
reliable survey of arsenic in groundwater was carried out under DPHE’s
Groundwater Studies for Arsenic Contamination in Bangladesh project in 1998
and 1999. The summary statistics from their national survey (excluding the
Chittagong Hill Tracts) are given below in Table C8.3.l and they represent the
least biased assessment of the groundwater affected by arsenic contamination.
However, because the population density is not evenly distributed across the
country, these percentages cannot be directly transformed into population
affected.
Table C8.3.1: Frequency Distribution of Arsenic
Concentrations in Groundwater
Concentration Class (mg/l) |
No in Class |
% of Wells in Class |
Cumulative No. of Exceeding |
Cumulative % Exceeding |
0-0.005 |
1838 |
52.0% |
3534 |
100.0% |
0.005-0.010 |
209 |
5.9% |
1696 |
48.0% |
0.010-0.050 |
606 |
17.1% |
1487 |
42.1% |
0.050-0.200 |
565 |
16.0% |
881 |
24.9% |
0.200-0.500 |
252 |
7.1% |
316 |
8.9% |
0.500-1.000 |
61 |
1.7% |
64 |
1.8% |
>1.000 |
3 |
0.08% |
3 |
0.08% |
All Samples |
3534 |
100% |
|
|
Source: Groundwater Project for Arsenic Contamination in
Bangladesh project and http://www.bgs.ac.uk/arsenic.
C8.3.2 Comparative Analyses
The DPHE study showed there are problems in measuring the concentration
of arsenic with accuracy even in a laboratory, which
need to be recognized when evaluating survey results. This shows up most
clearly when the results of tests carried out in DPHE
laboratories are compared with those carried out on the same samples in the
UK, as is done in Table C8.3.2 below. This shows a statistically significant
relationship that indicates the DPHE laboratory results overstate the
concentration of As by 25% on average. This does not greatly change the
findings about whether a particular well is harmful to health or not.
However, differences can be large at higher concentrations.
Table [sic] C8.3.2: Comparison of DPHE and BGS Analyses (Click
on the table for a larger view)
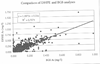
The differences are particularly important when reviewing the
the occurrence of As in the deep wells, As in Table 8.3.3 below. Whereas DPHE
results indicate a proportion of deep wells (>200m deep) exceed Bangladesh
standards, this is not confirmed by BGS control tests.
C8.3.3.Comparison of Contamination with Depth, DPHE
and BGS (Click inside for full view)
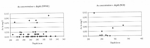
In the initial stages, it was suggested that testing had been
concentrated in areas where arsenic was known to occur, a procedure that would
lead to an over-estimation of the extent of the problem The plot in Table C8.3.4
below shows that this was not the case, as there was no meaningful relationship
between the number of tests per Thana and the proportion found positive.
Table C8.3.4: No of Tests V. Proportion Proving Positive
C8.3.3 Regional Distribution of Arsenic in Groundwater
The principal source of information on the distribution of arsenic comes
from the surveys and data compilations of the Groundwater Studies for
Arsenic Contamination project (DPHE, 1999). From the perspective of human
exposure, the most useful method of representing the geographical
distribution of arsenic is percentage of wells that exceed the drinking
water standard. In this form it is possible to combine laboratory data with
field kit results to access a much larger database. Combining this data on
l8,000 tests in over 3,000 unions with the NRWD GIS map of unions, it has
been possible to produce a probability Of arsenic occurrence across the
country as shown in the four maps in Figure C8.3.1. The percentage of wells contaminated was assigned to the centre point of the
union and then converted to a smooth surface using the inverse
distance-weighted technique.
The maps in Figure C8.3.l show the probability of exceeding the four
threshold concentrations of 0.01lmg/l (the WHO guideline value), 0.05mg/l
(the current drinking water standard), 0.20mg/l and 0.40mg/l. No
depth separation of wells has been applied in this map, but, as described
below, the deep aquifer system is almost completely uncontaminated. If a
lower drinking water standard (e.g. 0.01mg/l) is adopted the affected area
will expand considerably. On the other hand, the areas in which extremely high arsenic concentrations occur are much
more restricted, pointing the way to prioritizing interventions.
Figure C8.3.1 shows that arsenic-contaminated groundwater
may be found in most parts of the country but is strongly concentrated in the
South West, South East and North East. In large parts of the South East, more
than 70% of the wells are contaminated. In the case of Hajiganj upazila of
Chandpur District, Jakarya et al (1998) tested all 12,000 wells and
found that 93% were contaminated. There is currently very little data on the
occurrence of arsenic in the Chittagong Hill Tracts, which are geologically
very different from the areas tested.
The picture presented in Figure C8.3.2, which shows the
occurrence of arsenic in wells deeper than 200m, is very different. Although
far fewer deep tube wells have been tested, it is clear that the risk of
contamination at this depth is much lower.
The regional patterns of arsenic distribution is closely
related to its origin by reduction of iron oxy-hydro xides and has been
described in detail by DPHE (999), and the key features are described only in
summary here:
Arsenic occurs in the catchments of
all major rivers of Bangladesh. This demonstrates the existence of multiple
source areas.
Aquifers beneath the hills of greater
Sylhet and terrace areas (Barind and the Madhupur Tracts) are virtually free
of arsenic contamination. These aquifers are formed of older sediments from
which arsenic has either flushrd out or has been immobilized.
Of the major rivers, the Brahmaputra
and Teesta floodplains are least affected, while Meghna floodplains are worst
affected.
Over large parts of North west and
North Central Bangladesh and the Chittagong Coastal Plain the probability of
wells being affected by arsenic is less than by 5%.
Arsenic is far less likely to occur
in aquifers less than 200m.
Figure C8.3.1:Probability of Arsenic Exceeding Threshold
Values (Click on the image for a better view)
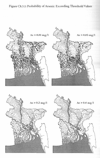
Figure C8.3.2: Arsenic in Wells with Depth Greater than
200m (Click the inside for a larger view)
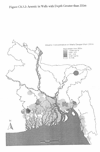
C8.3.4 Local Variation of Arsenic in Groundwater
The preview section described the systematic regional
scale variation of arsenic. However, within file affected areas studies have
shown striking and often systematic variations of arsenic concentrations on
a scale of hundreds of metres to a few kilometres related to specific
geological features. These variations take three main forms. First, at the
boundaries of older units such as the Madhupur and Barind Tracts are sharp,
predictable and sometimes extreme (DPHE, 1999). Sometimes within the
floodplains the patterns of distinct sedimentary features such as buried
channels or ox-bows can be identified in the arsenic maps (Safiullah, 1998;
AAN, 1999). These features ate mappable and represent distinct contaminated
and uncontaminated sections of aquifer, but they are not predictable from
surface expressions. In other cases (eg Burren, 1998), the patterns could be
related to mapped soil associations. At a larger scale, distinct higher or
lower probabilities of contamination can be associated with different
floodplain units (DPHE, 1999) and can be used for prioritizing surveys, but
it is not clear how reliable the boundaries of these units are for
predicting the occurrence of contaminated wells.
C8.3.5 Variations with Depth and Time
The probability of wells being contaminated is strongly
related to the depth at which they are screened. The probability of
exceeding the drinking water standard in the upper aquifer system is high,
but as shown in Table C8.3.5, in wells screened at lower levels, the risk
from arsenic is minimal. There has been some controversy (Chowdhury et
al, 1999) as to whether arsenic increases or decreases with depth.
However, as shown below and elsewhere, there is evidence that an increase in
depth of screening is related to a sharp
initial increase in the occurrence 0f contamination
followed by a gradual decease.
Table C8.3.5 Arsenic Occurrence in Groundwater by
Depth of Well
Depth Range |
No of Wells |
No >0.01mg/l (WHO Standard) |
No >0.05mg/l (BWDS) |
No >0.20mg/l |
Maximum (mg/l) |
<10m |
62 |
32(52%) |
15(24%) |
2 |
0.264 |
10-40m |
2012 |
899(45%) |
573(28%) |
242 |
1.090 |
40-100m |
1006 |
449(45%) |
248(28%) |
68 |
1.660 |
100-150m |
123 |
90(73%) |
42(34%) |
4 |
0.262 |
>200m |
38 |
6(16%) |
1(3%) |
0 |
0.059 |
Total |
288 |
9(3%) |
2(1%) |
0 |
0.108 |
|
3529 |
1485(42%) |
881(25%) |
316 |
- |
Source: Groundwater Studies for Arsenic Contamination in Bangladesh
It is clear from the table, in terms of both the
probability of contamination and the maximum probable concentration, that
well depth provides a clear basis for an arsenic avoidance strategy, at
least in the short to medium term. Nevertheless, it is also
important to note that in the DPHE survey 58% of the wells have
concentrations below the WHO guideline and 75% of them conform to
the current drinking water standard. Therefore there is no
basis for a general abandonment of the shallow aquifers, again at least in
the short to medium term. Figure C8.3.3 compares the depth-profile of the
probability arsenic exceeding 0.05mg/l with the typical depth ranges of
different types of wells, showing how different technologies are
differentially exposed to arsenic.
Figure C8.3.3 also highlights a regional anomaly in
Sunamganj where, due to the extreme rates of tectonic subsidence (4mm/yr),
arsenic-affected aquifers occur deeper than elsewhere in the country.
A certain note of caution should be observed regarding the
safety of deep aquifers. Wells deeper than 200m have only been extensively
tested in the coastal area. The extension and safety of such deep aquifers in
inland areas should be proven and should not be assumed. The question of
sustainability of pumping from deep aquifers is addressed below.
Despite an obvious lack of long-term monitoring data DPHE
(1999) have deduced that the proportion of wells that exceed the drinking
standard plus concentrations of 0.10, 0.15
and O.20rng/l) increases with age up to an age of eight to ten years. In the
long-term, concentrations of arsenic should decline as the stock of arsenic in
the sediments is depleted. DPHE (1999) supported by modeling studies by
Cuthbert (1999) attribute this to slow leakage from overlying aquitards. Their
practical conclusion is that ensuring a distance of 10m or more between the
well screen and an overlying aquitard massively reduces the risk of delayed
contamination. Unfortunately, it appears to he common (and understandable)
practice to screen at the top of the first available aquifer. The question of
seasonal variations of arsenic concentration is uncertain. AAN (1999) have
certainly reported differences between seasons, hut have not clearly proven
seasonal fluctuations. Such fluctuations are possible, especially in shallower
wells and where pumping is most intense. The Eighteen District Towns Water
Supply Project (18DTP, 2000) periodically monitored selected wells from 1996
to 1999, but reported no consistent trend or pattern. Ahmed (2000) reports
that nested monitoring wells installed in three Thanas under Phase II of the
Groundwater Studies for Arsenic
Contamination project showed no significant changes over seven months of
monitoring except for a rise in the first few weeks after installation. The
latter concentration has obvious implications for the testing and
commissioning of new wells.
Figure C8.3.3 : Depth Distribution of Arsenic in Groundwater (Click on
the Figure for a larger view)
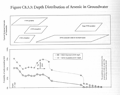
C8.4 Arsenic in Water Sources
C8.4.1 Arsenic in surface Water
Arsenic contamination in Bangladesh is principally a
problem in groundwater and it is often
stated that surface water is safe from arsenic. However, concentrations up
to 0.5mg/l (500ppb) have been
reported from a partially dried up river in Meherpur (Burren, 1993). While this is believed to be an exceptional
ease, it demonstrates that surface water cannot be assumed to be safe from
arsenic. It is anticipated that arsenic contamination of surface water is
most likely in ponds or in relatively stagnant water bodies that are fed by
groundwater flow. This is likely to be a seasonal phenomenon, occurring in
the dry season. The occurrence of arsenic in some surface waters also raises
the possibility of arsenic uptake by fish.
C8.4.2 Arsenic in Dug Wells
It was noted earlier that the risk of arsenic contamination is low in
very shallow groundwater. It has been widely reported that dug wells are
safe from arsenic. Dr. Dipankar Chakraborty reports that analyzing more than
100 dug wells from West Bengal and Bangladesh and that none exceeded
0.05mg/l. The problem with dug wells is not arsenic, but the risk of
diarrhoeal disease.
C.8.4.3 Arsenic in Tube wells in the Shallow or Main Aquifer
The majority of private water supplies are obtained from
dried tube wells between 10 and 100 metres deep. There are no reliable
figures but there may be between eight and eleven million such wells the
country. These sources pose the principal threat to public health from
arsenic. Nevertheless, the majority (>70%) of such wells have arsenic
contamination levels of less than 0.05mg/l and
they also pose a low risk of diarrhoeal disease. As noted above arsenic
concentrations may increase over time (most probably in wells screened very
close to aquitard layers). The continued safety of presently
uncontaminated wells is an important water management issue.
C8.4.4 Arsenic in Deep Tube wells
Because of the differing nomenclature in irrigation and
water supply circles, the term ‘deep tube wells’ regularly causes major
confusion. Reports from BADC that arsenic occurs in deep tube wells can
cause confusion because BADC refer to wells with depths in the range 150 to
350 feet (50 to 110cm). In addition, BADC set screens at depths of 50-80m,
thus drawing water from the shallow aquifer, which is known to be
contaminated.
Here, the term is used to refer to wells screened in
aquifers deeper than 200m. However, it is strongly recommended that depths
are always quoted when this subject is discussed. Most wells screened below
200m have arsenic concentrations below detection limits and less than 2%
exceed the Drinking Water Standard, and even then only by a small amount.
The distribution of arsenic in wells deeper than 200rn is shown in Figure
C8.3.3, from which it is clear that the majority conforms to even the
proposed new US standard.
C8.4.5 Changes over Time
The question is often asked if the arsenic map' will
change in a few years' time. Of course, some change will occur as more data
are collected and uncertainty is reduced. It is possible, but not at all
certain, that there will be an increase in the percentage of wells
contaminated in the badly affected areas, however, it seems unlikely that
new areas of contamination will emerge. The greatest change that can be
anticipated in the ‘arsenic map’ will come from a change in the
regulations governing drinking water standards and not from any hydrological
processes.
C8.5 Impact of Arsenic on Drinking Water Supplies
C8.5.1 Population Exposed
A simple assessment of the population exposed to hazardous levels of arsenic
was made using the 1991 census and the National Arsenic Survey of the
Groundwater Studies for Arsenic Contamination project carried out in 1998 and
1999. That survey is the only one that has a large, evenly spaced
and reliable arsenic concentration data over the whole country (excepting
the Chittagong Hill Tracts). The arsenic distributions shown earlier in'
Figure C.8.3.2 have been combined with the population distribution to
estimate the exposed populations at the 10, 50, 200 and 400ppb
levels. The estimates are made for the year 2000 assuming a population
growth rate of 2.2% per year and that 95% of the population draw their
water from groundwater supplies. No explicit account has been taken of the
difference in arsenic between the deep and shallow aquifers, however, the
survey design was stratified to reflect the importance of deep and shallow
aquifers in each upazila. The results of the assessment are given
tnTahleC8.5.1 below.
Table C8.5.1. Estimated Population Exposed to Arsenic
in Drinking Water
Arsenic Concentration |
Exposed Population in 2000 |
>10ppb
>50ppb
>200ppb
>400ppb |
47.5M
29.0M
10.3M
0.9M |
Total Population |
125.0M |
Notes: Table excludes the three CHT Districts due to lack of arsenic data.
The distribution of population exposed is somewhat different
to the arsenic distribution itself because the most affected areas of the south
- east of the country also correspond to the areas of highest population
density.
C8.5.2 Options for Avoidance or Treatment
Preventing exposure to arsenic in drinking water by
providing arsenic-free water supplies is the essential requirement for the
improvement of health in the affected areas. There are three basic
approaches:
The distribution of population exposed is somewhat different
to the arsenic distribution itself because the most affected areas of the south
- east of the country also correspond to the areas of highest population
density.
C8.5.2 Options for Avoidance or Treatment
Preventing exposure to arsenic in drinking water by
providing arsenic-free water supplies is the essential requirement for the
improvement of health in the affected areas. There are three basic
approaches:
The distribution of population exposed is somewhat different
to the arsenic distribution itself because the most affected areas of the south
- east of the country also correspond to the areas of highest population
density.
C8.5.2 Options for Avoidance or Treatment
Preventing exposure to arsenic in drinking water by
providing arsenic-free water supplies is the essential requirement for the
improvement of health in the affected areas. There are three basic
approaches:
-
Treatment of arsenic
contaminated groundwater;
-
Develop arsenic-free
groundwater sources; or
-
Develop surface water sources such as
rivers, ponds and rainwater. All three approaches have relevance in
different settings, and all have some difficulties. Hydrological and
climatic factors obviously constrain the technical selection of
methods, while the scale (eg household, community, municipal) of the
operation will determine their social and economic suitability.
Detailed discussion of the selection of methods is given elsewhere
in the DDS report. The choice of method must also be considered in
relationship to different drinking water standards. It is known that
common methods such as aeration and coagulation-filtration all
effectively reduce arsenic to around 0.05mg/l but not to 0.01mg/l.
C8.6 Trends of Arsenic in Groundwater
Following the description of the
occurrence of arsenic earlier, a number of sustainability questions arise which can be divided
into those affecting the shallow or main aquifer (i.e. wells less than
100-120m deep) and those in the deep aquifers (wells more than 150-200m
deep). Important questions affecting the use of the shallow aquifers are:
-
Will presently
uncontaminated aquifers become contaminated in the future? This relates
particularly to the extensive uncontaminated aquifers such as the Dupi Tila
sands beneath the Madhupur and Barind Tracts.
-
Will uncontaminated
wells in partially contaminated aquifers become contaminated in
the future? There are many cases of sharp lateral variations of
arsenic concentrations within villages, giving rise to obvious
fears of lateral migration of arsenic.
The issues relating to the sustainability of abstraction from
the deep aquifers are:
-
Will wells in deep
(>150-200m) aquifers that are overlain by contaminated aquifers
become contaminated by downward leakage of arsenic? This is of
greatest concern where increased pumping from the deep aquifer is
accompanied by the abandonment of existing wells in the upper
aquifer. This is usually a local problem, where the degree of risk
depends on the intensity of abstraction and the properties of the
intervening strata.
-
Will increased pumping
from deep aquifers induce saline intrusion in the coastal areas?
Unlike the above concern, the risk results from the cumulative
effects of deep well abstraction over a large region and will
impact most on water supplies closest to the coast.
The above four questions may then be reformulated as three
hydrogeochemical questions:
(a) Will conditions in uncontaminated aquifers
change sufficiently to mobilize from sediments where arsenic is
presently stable in the solid phase?
(b) At what rates will water, salinity (a
conservative tracer) and arsenic migrate laterally and vertically
through the aquifers?
(c) If arsenic is drawn into presently
uncontaminated layers, will it remain in solution or be adsorbed on
to the solid phase?
Regarding question (a), given that the main driving force for
mobilization of arsenic is decay of organic matter in the sediment and this is a
finite quantity, the answer is likely to be ‘no’. Only in the exceptional
circumstances such as infiltration of landfill leachate is groundwater likely to become so reducing as
to mobilize arsenic from the sediment.
With reference to question (b), tile migration of
arsenic is constrained by the rates of water
movement and by the effects of adsorption. Both have been studied by
numerical modeling by DPHE (1999) arid Cuthbert (1999). There is currently
significant uncertainty concerning the quantification of sorption
parameters for arsenic. Both studies show even without including the
effects of sorption that, under realistic scenarios, arsenic will not move
more than a few meters a year laterally or vertically. With only modest sorption, the migration rates drop to less
than a meter per year.
Uncertainty concerning sorption parameters prevents a
precise answer to question (c). However, the direction of the process is
clear and it will operate to some degree to slow down arsenic mitigation.
C8.6.1 Shallow Aquifers
Extensive areas presently free of arsenic are expected to
remain so. The reasons are twofold. In the coarser aquifers (such as beneath
the Brahmaputra and Teesta floodplains), little arsenic and
organic matter accumulated at the time of deposition. In the older aquifers
(such as the Madhupur and Barind Tracts and Dhaka City), arsenic has
either been flushed out or is combined with more stable iron minerals (such
as hematite or goethite) and the aquifers' redox conditions are apparently
not sufficiently reducing to mobilize any arsenic that may he present in the
sediment.
The future trends of arsenic in partially contaminated
areas are more difficult to predict. The modeled migration rates and inferred
increases over time strongly suggest that vertical leakage is the critical
factor, leading to delayed contamination of tube wells. This may be expected
to continue if well design practice is not changed. On the other hand, the
risk of contamination due to lateral migration of more than a few tens of
meters is small. For the 25-year planning horizon of the Plan, the probable
upper limit of lateral migration is considered to be of the order of 50
meters, and vertical migration smaller still. Further research is needed to
provide better estimates of migration rates for arsenic, particularly where
inter-aquifer movement is involved. Sufficient is known to be confident of no
very rapid movement, and some research is underway on this subject, but the
results should be reviewed after a few years.
C8.6.2 Deep Aquifers
There is very little arsenic contamination in aquifers below 150-200m. The main and deep aquifers
are usually separated by lower permeability strata. The vertical hydraulic
resistance and anisotropy of the intervening strata suggest that the
migration of arsenic from contaminated shallow aquifers would occur in a
period of not less than decades and probably centuries. If the probable, but
poorly quantified, effects of sorption are included, it is like~ that the
deep aquifer system offers the prospect of permanent or at least very long term, sources of drinking
water that is free of bot arsenic and bacterial contamination.
Use of the deep aquifer system will be of most importance
in the coastal regions of Bangladesh. There the risk of arsenic must be
assessed in parallel with that of salinisation. In this hydraulic situation,
the critical issue is the source of water that replaces the water pumped from
deep wells. While existing performance in the most heavily abstracted areas
such as Khulna City is encouraging, it is impossible at present to say
how much more abstraction can be supported. There is a need for a detailed and
systematic assessment of the resources of the deep aquifer system to be
implemented in parallel with the water supply development.
C8.7 Arsenic, Irrigation and Agriculture
The question as to whether there is a causal relationship
between arsenic and tube well irrigation was addressed earlier, and it was
concluded that here is no such causal link. However discussion of the
inter-relation of arsenic and irrigated agriculture must he extended to ask
whether arsenic in irrigation water affects the growth of crops, and whether
arid how arsenic in irrigation water or soil might accumulate the food chain?
C8.7.1 Arsenic in Food grain
There are few analyses on arsenic in food grain in
Bangladesh. However, no reports are available of analyses of rice or
wheat containing arsenic at levels of health significance. Most analyses show
arsenic below detection limits. 0f the food grains rice has the most potential
to take up arsenic due to the anaerobic root zone (Chappell, 2000). No
comprehensive study has been carried out, although a study is underway at
CSIRO in Australia and results are expected in late 2000. The CSIRO study will
make a survey of arsenic in vegetables and crops, plus their associated soils
and water sources across Bangladesh and West Bengal. The long-term objective
is to integrate the arsenic-crop-soil-water-medical relations
to develop a landscape risk assessment model.
A case study of arsenic at two shallow tube wells in Faridpur by Hasan
(1998) is described in Table 8.7.1 below. When considering the total arsenic concentrations in soil, it should be
remembered that the arsenic may be present from the time of deposition of the
sediment and has not necessarily been added from irrigation water. The high
arsenic concentrations in soil at the new well with a low arsenic
concentration in groundwater make this clear. Chappell (2000) reports that an
arsenic content of 10mg/kg is typical for soils world-wide. Certainly the
arsenic in the Devinagar soi1 profile could not have been accumulated from
irrigation water in the single year of operation. This therefore makes it
uncertain as to whether there has been significant accumulation of arsenic in
the Shasa profile. The major uncertainty
in the processes is the quantity of arsenic removed from the system (to the
atmosphere) by biomethylation under the action of fungi, yeast and bacteria.
Table C.8.7.1 Arsenic Analyses from Irrigated Rice Production in Faridpur
Sample Type |
Range of Arsenic Concentrations (mg/l or mg/kg) |
|
Well-1 (Shasa Est. 1989) |
Well-2 (Devinagar Est. 1998) |
Tube well |
0.246 (Fe - 8.8) |
0.083 (Fe - 3.5) |
Field Channel |
0.198 (Fe - 4.5) |
0.075 - 0.080 (Fe - 3.0) |
Soil Samples |
6.6 - 10.6 |
5.3 - 8.0 |
Rice Root |
224 |
162 |
Rice Shoot |
2.70 |
Nd |
Rice Leaves |
4.35 |
4.25 |
Rice (not paled) |
0.00 |
0.00 |
Rice (paled) |
0.00 |
0.00 |
Source: Hasan (1998)
At the two STW sites, no arsenic was found in the rice, but high arsenic concentrations
were found in the root. This is consistent with the physiology of rice and
other plants where the distribution of arsenic is in descending order from
root to stem to leaf and edible parts (Huang, 1994). Also the results from
the 'Devinagar' site suggest the possibility that arsenic in the root might
be derived from the soil. There is also evidence that arsenic concentrations
are being reduced by aeration along with iron between the well and the
field.
The important conclusion from Hasan’s study is that
irrigated rice plants may be exposed to sources of arsenic in soil and water
without transferring the arsenic to the directly edible parts of the plant.
However, the question as to whether the cultivation of irrigated rice is
sustainable is fundamental to the formulation of the NWMP and any serious
uncertainty in this area must be explicitly addressed.
C8.7.1.1 Arsenic in Vegetables and Fruits
Professor Imamul Huq of Dhaka University has shown that arsenic may
accumulate in the edible parts of leafy vegetables. Although these are not
specifically water management issues, they are extremely important and
require urgent investigation. It is expected that the CSIRO will provide a
major insight on these issues by the end of 2000.
C8.7.1.2 Other Pathways
In addition to direct consumption of arsenic in fruit, grains and vegetables, it is wise to
consider whether there are indirect exposure pathways. It was noted earlier
that there is slight accumulation in rice straw which is used as feedstuff for
livestock;
indeed any other fodder grown on arsenic contaminated soils are potential
pathways of exposure. In
particular it is important to check for mechanisms of bioaccumulation in
different parts of the animals' anatomy. No data information has been
identified from Bangladesh on this subject. However, the CSIRO study noted
above includes animal studies of the bioavailability of arsenic from
vegetables and it also quantifies ingestion of arsenic from processed foods
such as cooked meat and milk.
Another potential exposure pathway is consumption of
fish kept in groundwater fed ponds. The Noakhali Aquaculture Extension
Project has analyzed tissue and
liver from Common Carp, Gras Carp, Mrigal, Golda and Ruwi. Arsenic
ranged up to a maximum of 2.0mg/kg of dry weight. The samples also
contained between 0.11 and 0.66mg/kg (of dry weight) of mercury. A
significant part off the arsenic found in fish is usually in less toxic
organic forms, and the health significance of arsenic in fish is yet to
evaluated.
C8.7.1.3 Yield Reduction in Rice
FAO Irrigation and Drainage Paper 29 (Water Quality for
Agriculture) reports that arsenic is toxic to rice, and causes a reduction
of yield, at the same levels as it is toxic to humans in drinking water
(0.05mg/l). Based on studies
in Japan, Huang (1994) notes
that although there is a weak correlation with total arsenic content there
is a strong correlation with soluble arsenic in the soils. The factors
controlling crop yields are many and complex and some factors rarely
dominate yields under normal conditions. The effect of arsenic on rice
yield remains an area of uncertainty for water management, and it is
believed (Chappell, 2000) that yield reduction in
rice occurs at concentrations below those resulting in visible symptoms of
photo-toxicity.
Arsenic is also photo - toxic to soybean, cowpeas and
oats.
C8.7.2 Spatial Distribution of the Arsenic Hazard in
Agriculture
Reference to Figure C8.3.2 shows that most of the areas
of intensive ground water irrigation ( in the greater Districts of Dhaka,
Mymensingh, Bogra and Rangpur) do not correspond to areas of severe
arsenic contamination. The areas of potential risk are most in the
Kushtia-Jessore and Chandpur-Brahmanbaria regions.
C8.7.3 Preliminary Assessment and Research Needs
Groundwater with potentially toxic levels of arsenic is
being used as a source of irrigation water in significant areas of
Bangladesh. Although no conclusion on the scope for yield reduction can be
given at this time, some preliminary conclusions can be given on the scope
for arsenic accumulation in food grains and vegetables.
-
Analyses for rice grains give little indication of bio -
accumulation of arsenic.
-
Arsenic in food - stuffs is usually less toxic organic
forms.
-
There is evidence that rice
grown with contaminated irrigation water does not accumulate arsenic in the
grain.
-
Arsenic in irrigation water may reduce the
yield of rice.
-
Arsenic is accumulated by some
vegetables.
-
There is shortage of relevant
data.
Thus it might be concluded that there is no direct
evidence to support a change in policy in the use of groundwater for
irrigation from contaminated aquifers at this stage. However, there are
reasons for caution in acting on this conclusion. First, the present
data-base is too small to give a high degree of confidence. Second, the
possible consequences of arsenic accumulation through long-term irrigated
agriculture are simply too important to be left as a matter of scientific
judgment. Intensive studies of the present situation and initiation of
long-term monitoring are essential. Two lines of study are recommended
below.
Long-term (ten to 20 years) monitoring of crop, soil
and water monitoring should be established at say ten to 20 sites
under differing agro-ecological conditions. The monitoring would
concentrate on changes in the water and arsenic balances using the tube
well catchments as a unit of measure. This is considered essential, but
unfortunately will take many years to reach a definite conclusion, and a
more rapid conclusion is needed..
DPHE (1999) used a cross-sectional sampling technique
to infer with a high degree of confidence the
temporal changes in arsenic concentration at wells. Beyond any reasonable
doubt, arsenic contaminated irrigation water has been used in some areas
for 20-30 years. If accumulation is a significant problem, it should
already be apparent at older schemes. A stratified or randomized survey
can therefore be designed to collect water, soil and crop samples at wells
of different ages and with varying arsenic concentrations. Arsenic
concentrations can therefore be tested against the cumulative loads of
both arsenic and water applied. The survey may also be aggregated to
identify the influence of soil type and climate. If the sample site is
large enough, it should be possible to deduce the effect of arsenic
concentration on crop yield.
8.7.3.1 Other Groundwater Quality Issues
A summary of analyses
of 3538 evenly spaced samples is given in Table C8.7.2 below, taken from
the GSAC National Survey. In addition to arsenic the frequent
exceedances of the health related parameters boron and manganese and
occasional exceedances of barium and chromium. Although not-health
related, iron exceeds that national standard at 4l% of wells.
Table C8.7.2 : Summary of Groundwater Quality Baseline
Survey
Parameter |
Drinking Water Standard |
Wells Exceeding |
Maximum Concentration |
Comment |
No |
% |
(mg/l) |
Ratio to Standard |
Arsenic |
0.05 |
881 |
25% |
1.66 |
33 |
WHO guideline is 0.01 mg/l |
Boron |
0.5 |
170 |
4.8% |
2.19 |
4 |
New WHO guideline since 1998 |
Barium |
0.7 |
10 |
0.3% |
1.36 |
2 |
|
Chromium |
0.05 |
8 |
0.2% |
0.72 |
14 |
|
Copper |
2 |
0 |
- |
0.14 |
- |
|
Iron |
2 |
1449 |
41% |
61 |
31 |
Not health related, but WHO aesthetics guideline is 1.0, and 0.3
preferred for public supply |
Manganese |
0.5 |
1246 |
35% |
9.98 |
20 |
Aesthetics guideline |
|